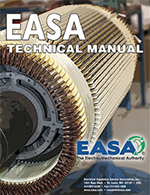
Revised September 2022
The EASA Technical Manual is the association's definitive and most complete publication. It's available FREE to members in an online format. Members can also download PDFs of the entire manual or individual sections.
VIEW & DOWNLOAD